Reading Notes
for Chapter 17
These are Dr. Bodwin's reading notes for Chapter 17 of "Chemistry
2e" from OpenStax.
I am using a local .pdf copy that was downloaded in May 2020.
Chapter
Summary:
Chemistry is almost all about the electrons and nothing shows that more
clearly than redox chemistry. Everyone uses redox chemistry every day.
Every battery is redox chemistry. Most metalloenzymes work because of
redox chemistry. It's both a ubiquitous and specific field.
Redox also offers us a number of well-defined rules and processes. If
you like rules, mnemonic devices, and step-by-step processes, you have
found a comfortable home in redox chemistry.
Oxidation
Numbers:
Oxidation numbers compare the number of protons to the number of
electrons associated with an atom. For monoatomic ions and atoms,
"oxidation number" is the same as "charge", but for anything polyatomic
we have to be a little more careful.
IMPORTANT POINT: oxidation number is a characteristic of each "atom" in
a polyatomic species. A molecule or polyatomic ion does not have an oxidation number, it
has a charge.
Time for some rules...
Assigning
Oxidation Numbers (Rules):
- For neutral elements uncombined with other elements, the
oxidation number is zero
- For monoatomic ions, the oxidation number is equal to the charge
- Oxygen is almost always oxidation number -2 except for O2
(see
rule 1) and peroxides (Ox# = -1)
- Hydrogen is almost always oxidation number +1 except for H2
(see
rule 1) and hydrides (Ox# = -1)
- The sum of the oxidation numbers in a polyatomic ion or molecules
must equal the charge on the polyatomic ion or molecule.
The rules work for most ionic compounds, and they are relatively easy
to use. Sodium sulfate is 2 sodium ions and a sulfate ion. The sodium
ions are monoatomic, so the oxidation number on each sodium ion is +1
(see Rule 2). The sulfate ion has an overall -2 charge. If each of the
four oxygens has oxidation number -2 (Rule 3) for a sum of -8, then the
sulfur must have oxidation number +6 because the sulfate polyatomic ion
has a total charge of -2 (Rule 5).
When we're dealing with a more structural
formula, the rules sometimes lead us astray. What about sodium acetate?
The sodium is still +1, but what about the C, H, & O in the acetate
ion? If we assume the hydrogens are all +1 (Rule 4) and the oxygens are
all -2 (Rule 3), then the two carbons are each oxidation number zero.
(check that...) Having an oxidation number of zero is fine, BUT in this
case we are assuming that both carbons are the same oxidation number.
With one carbon bound to 3 hydrogens and the other bound to 2 oxygens,
that seems like it might not be a great assumption. When "the rules"
don't seem to work, we can look at a chemical structure and use that to
assign oxidation numbers in a process similar to that use for formal
charge. Assigning Oxidation Numbers in Lewis Structures
NOTE: For anyone who will be taking Organic Chemistry, assigning
oxidation number (and formal charges) from structures is a very useful
tool for understanding organic chemical reactions. Assigning oxidation
numbers from structures also has the benefit of being able to assign
oxidation numbers to a small part of a large molecule without assigning
every atom within the structure.
Oxidation
and Reduction:
Oxidation and reduction describe the process of moving electrons
between chemical species. These are couple process. You can't have
reduction without oxidation, and you can't have oxidation without
reduction. If you find one, look for the other. {Exception: oxidation
and reduction can be driven by electricity where an electrical current
is used to either supply or remove electrons, but this isn't what we're
talking about here.}
How do you keep them straight? Here's where some mnemonic devices come
in handy.
Oxidation
Is Losing
electrons
Reduction
Is Gaining
electrons
OIL-RIG
Or if you prefer a non-petroleum-based option:
Losing
Electrons
is Oxidation
Gaining
Electrons
is Reduction
LEO the lion says GER
Balancing
Redox Reactions:
Many redox reactions can be balanced by trial and error. Just remember
that the total charge on the reactant side has to equal the total
charge on the product side. Reactions involving only monoatomic metal
ions and their elements can usually be balanced by trial and error.
Some redox reactions can be excpetionally difficult to balance by trial
and error, so it is helpful to have a process to balance them. Your
textbook has a set of steps on pages 898-901 that are pretty commonly
used and tend to work well, but I prefer a slightly modified approach
described here:
https://chemistryingeneral.blogspot.com/2012/04/balancing-redox-reactions.html
The benefits to this approach are: 1) it focusses on the electrons and
the transfer of electrons that takes place during a redox reaction; 2)
it offers a couple of "check points" along the way that help identify
problems before going through the whole process. Both processes work,
and as long as you do every step correctly, the process in your
textbook will produce the correct answer... with so many steps, it's
not hard to make a little msitake along the way, though, and my method
offers opportunities to catch those little mistakes before you go
through the whole process.
Whichever method you use, balancing redox reactions takes practice.
Practice, practice, practice!
Galvanic/Voltaic
Cells:
"Galvanic" and "voltaic" cells are the same thing. Different sources
prefer one over the other, so you'll run into both terms, but they mean
the same thing. I tend to use the term "voltaic cell" when I write or
speak.
This is a simplified diagram of a voltaic cell:
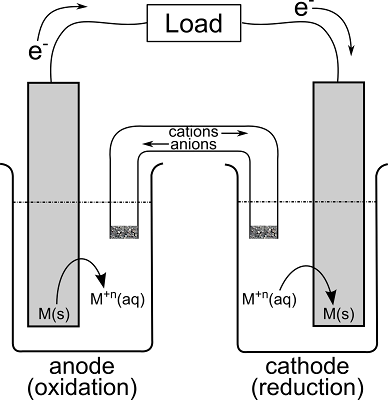
{https://chemistryingeneral.blogspot.com/2012/04/voltaic-cells.html}
The key to voltaic cells is keeping track of the moving charge, either
the moving electrons or the moving ions in the salt bridge. It helps to
always draw this diagram out the same way, with the anode on the left
and the cathode on the right (they're in alphabetical order than
way...} and the electrons in the external circuit flowing left to right.
Oxidation
takes place at the anode, reduction
takes place at the cathode.
If you go to a farm, you might see An Ox or a Red Cat to help you
remember this. Or you might remember that anode and oxidation both
start with vowels, cathode and reduction both start with consonants.
Cell
Notation:
This is one of the places where you get to use the "pipe" character on
your keyboard!! It's usually a shifted backslash and appears as a tall
vertical line, "|".
Standard cells are usually written as reductions... if you're pairing
two half-cells, one of them will have to be reversed to give an
oxidation. How do you decide which one to reverse? Well...
Cell
Potentials and Half-Cell Potentials:
A cell potential is a measure of the "load" in the above picture. If
the electrons are moving as shown
in the picture, the cell potential is positive. This is a spontaneous
voltaic cell. Rather than measuring every possible combination of
half-cells, we measure all of them relative to an accepted reference,
usually the Standard Hydrogen Electrode ("SHE").
Oxidation and reduction are opposite processes. If a reduction
potential (as measured relative to SHE) is know, changing the sign
yields the oxidation
potential for the opposite process.
This is another place where your textbook uses a very common pocess
that I don't always like. To figure out cell potential, your book uses
the equation:
E°cell = E°cathode - E°anode
That equation works. The reason I don't always like it is that you have
to remember which half is the cathode and which is the anode, and you
have to put them in the right order because subtraction is directional.
When I'm looking at cell potentials, I use the equation:
E°cell = E°reduction + E°oxidation
For the oxidation half reaction, change the sign of the standard reduction potential to get a standard oxidation
potential, then add them up. Mathematically, "change the sign" is the
same as "subtract", so these really are the same process, but
explicitly changing the potential of the oxidation reaction to an oxidation potential
makes my method more connected to understanding the processes that are
taking place and less about memorizing an equation. That's why I prefer
it. Either one will give you the same correct answer.
Non-standard Conditions and Equilibrium:
These are closely related to the discussions in the previous chapter
and are described well in your textbook. Using these relationships, you
should be able to interconvert between K°, ∆G°rxn, and E°cell.
Batteries and Fuel Cells:
These are some of the most obvious applications of redox chemistry.
Read them over and make sure you can identify half-reactions and
concepts.
Corrosion:
One of the most important reasons to study something like redox
chemistry is so that we can use it to our advantage. There are many
important investments that are made of metal, from cars to grain bins.
Corrosion destroys these investments, so it's worth considerable effort
to protect them from corrosion.
The two main ways to protect something from corrosion are:
- Anodic Inhibition
- When a metal corrodes ("rusts"), it is oxidized from elemental metal
with an oxidation number of zero to a metal cation with a postive
oxidiation number. Oxidation takes place at the anode, so what we are
trying to accomplish by anodic inhibition to to prevent the metal from
being an anode. This is most often done with some sort of coating (a
paint or oil or grease) that forms a barrier and prevents transfer of
eletrons and ions. In the picture above, if the anode is painted, the
electron and cation transfer that is required for the cell to work will
not happen. Anodic inhibition has the same effect as snipping the wire
that connects the two electrodes via the external curcuit... if we
break the circuit, the reaction cannot happen.
- Cathodic Protection
- This is a little more "clever" approach. Rather than just preventing
the metal from being the anode, in cathodic protection we force the metal to be a cathode
by connecting it to a more active metal. The more active metal serves
as the anode and is sacrificed to protect the metal of interest. This
is often used with things like grain bins; a large rod of zinc (or
another more active metal) is connected to the steel of the grain bin
and then pounded into the ground. The zinc rod corrodes while the steel
bin does not. These sacrificial anodes must be replaced from time to
time as they corrode. Another example of this is galvanized steel. This
is steel that has been coated with a thin layer of zinc metal to act as
both a coating (anodic inhibition) and a sacrificial anode (cathodic
protection) for the steel.
Electrolysis:
As mentioned above, a non-spontaneous redox reaction can be forced
by applying an electrical current. In the picture above, the "load" is
passively observing the flow of electrons in the extrenal circuit, but
if a source rather than a
load was connected to the external circuit, we could force the reaction
backwards, in the non-spontaneous direction.
Electrolysis is an important process for production of a variety of pure chemicals, but it can be expensive.
From a calculation perspective, let the units guide your problem
setup... Faraday's constant and electrical units such as amperes have
units that can help guide problem setup.
Return to ChemBits
General Chemistry Index.
All information on this page is produced by Jeffrey
Bodwin,
Copper Sun Creations, or curated from the attributed source.

This work is licensed under a Creative
Commons Attribution-NonCommercial-ShareAlike 4.0 International License.